BRIEF OVER VIEW OF FIBER OPTIC
CABLE ADVANTAGES OVER COPPER:
• SPEED:
Fiber optic networks operate
at high speeds - up into the gigabits
• BANDWIDTH:
Large carrying capacity
• DISTANCE:
Signals can be transmitted further
without needing to be "refreshed" or strengthened.
• RESISTANCE:
Greater resistance to electromagnetic noise
such as radios, motors or other nearby cables.
• MAINTENANCE:
Fiber optic cables costs much less to maintain.
In recent years it has become apparent that
fiber-optics are steadily replacing copper wire
as an appropriate means of communication
signal transmission. They span the long
distances between local phone systems as well
as providing the backbone for many network
systems.
Other system users include cable television
services, university campuses, office buildings,
industrial plants, and electric utility companies.
A fiber-optic system is similar to the copper
wire system that fiber-optics is replacing.
The difference is that fiber-optics use light
pulses to transmit information down fiber lines
instead of using electronic pulses to transmit
information down copper lines. Looking at the
components in a fiber-optic chain will give
a better understanding of how the system works
in conjunction with wire based systems.
At one end of the system is a transmitter.
This is the place of origin for information
coming on to fiber-optic lines. The transmitter
accepts coded electronic pulse information
coming from copper wire. It then processes and
translates that information into equivalently
coded light pulses.
A light-emitting diode (LED) or an
injection-laser diode (ILD) can be used for
generating the light pulses. Using a lens,
the light pulses are funneled into the fiber-optic
medium where they travel down the cable.
The light (near infrared) is most often 850nm for
shorter distances and 1,300nm for longer
distances on Multi-mode fiber and 1300nm for
single-mode fiber and 1,500nm is used for for
longer distances.
Think of a fiber cable in terms of very long
cardboard roll (from the inside roll of paper
towel) that is coated with a mirror on the inside.
If you shine a flashlight in one end you can see
light come out at the far end - even if it's been
bent around a corner.
Light pulses move easily down the fiber-optic
line because of a principle known as total
internal reflection. "This principle of total internal
reflection states that when the angle of
incidence exceeds a critical value, light cannot
get out of the glass; instead, the light bounces
back in. When this principle is applied to the
construction of the fiber-optic strand, it is
possible to transmit information down fiber
lines in the form of light pulses. The core must a
very clear and pure material for the light or in
most cases near infrared light (850nm, 1300nm
and 1500nm). The core can be Plastic (used
for very short distances) but most are made
from glass. Glass optical fibers are almost
always made from pure silica, but some other
materials, such as fluorozirconate,
fluoroaluminate, and chalcogenide glasses, are
used for longer-wavelength infrared
applications.
There are three types of fiber optic cable
commonly used: single mode, multimode and
plastic optical fiber (POF).
Transparent glass or plastic fibers which allow
light to be guided from one end to the other with
minimal loss.
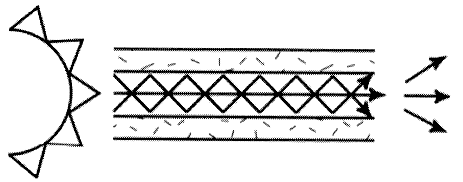
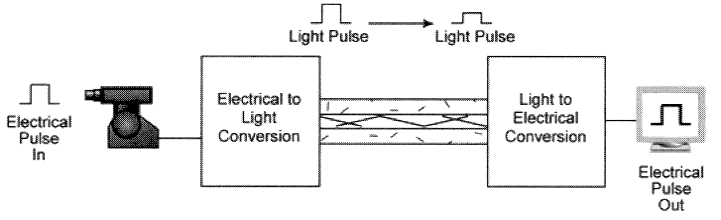
Fiber optic cable functions as a "light guide,"
guiding the light introduced at one end of the
cable through to the other end. The light source
can either be a light-emitting diode (LED))
or a laser.
The light source is pulsed on and off, and
a light-sensitive receiver on the other end of
the cable converts the pulses back into the
digital ones and zeros of the original signal.
Even laser light shining through a fiber optic
cable is subject to loss of strength, primarily
through dispersion and scattering of the light,
within the cable itself. The faster the laser
fluctuates, the greater the risk of dispersion.
Light strengtheners, called repeaters, may be
necessary to refresh the signal in certain
applications.
While fiber optic cable itself has become
cheaper over time - a equivalent length of
copper cable cost less per foot but not in
capacity. Fiber optic cable connectors and the
equipment needed to install them are still more
expensive than their copper counterparts.
Single Mode cable
is a single stand (most applications use 2
fibers) of glass fiber with a diameter of 8.3 to
10 microns that has one mode of transmission.
Single Mode Fiber with a relatively narrow
diameter, through which only one mode will
propagate typically 1310 or 1550nm. Carries
higher bandwidth than multimode fiber, but
requires a light source with a narrow spectral
width. Synonyms mono-mode optical fiber,
single-mode fiber, single-mode optical
waveguide, uni-mode fiber.
Single Modem fiber is used in many
applications where data is sent at multi-
frequency (WDM Wave-Division-Multiplexing)
so only one cable is needed - (single-mode on
one single fiber)
Single-mode fiber gives you a higher
transmission rate and up to 50 times more
distance than multimode, but it also costs more.
Single-mode fiber has a much smaller core
than multimode. The small core and single
light-wave virtually eliminate any distortion that
could result from overlapping light pulses,
providing the least signal attenuation and the
highest transmission speeds of any fiber cable
type.
Single-mode optical fiber is an optical fiber in
which only the lowest order bound mode can
propagate at the wavelength of interest typically
1300 to 1320nm.
jump to single mode fiber page
Multi-Mode cable
has a little bit bigger diameter, with a common
diameters in the 50-to-100 micron range for the
light carry component (in the US the most
common size is 62.5um). Most applications in
which Multi-mode fiber is used, 2 fibers are
used (WDM is not normally used on multi-mode
fiber). POF is a newer plastic-based cable
which promises performance similar to glass
cable on very short runs, but at a lower cost.
Multimode fiber gives you high bandwidth at
high speeds (10 to 100MBS - Gigabit to 275m
to 2km) over medium distances. Light waves
are dispersed into numerous paths, or modes,
as they travel through the cable's core typically
850 or 1300nm. Typical multimode fiber core
diameters are 50, 62.5, and 100 micrometers.
However, in long cable runs (greater than 3000
feet [914.4 meters), multiple paths of light can
cause signal distortion at the receiving end,
resulting in an unclear and incomplete data
transmission so designers now call for single
mode fiber in new applications using Gigabit
and beyond.
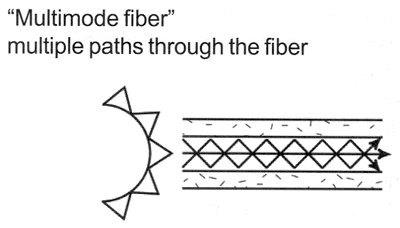
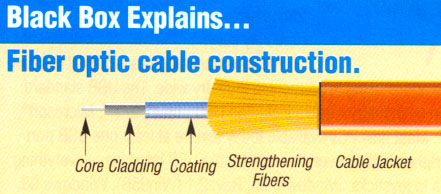
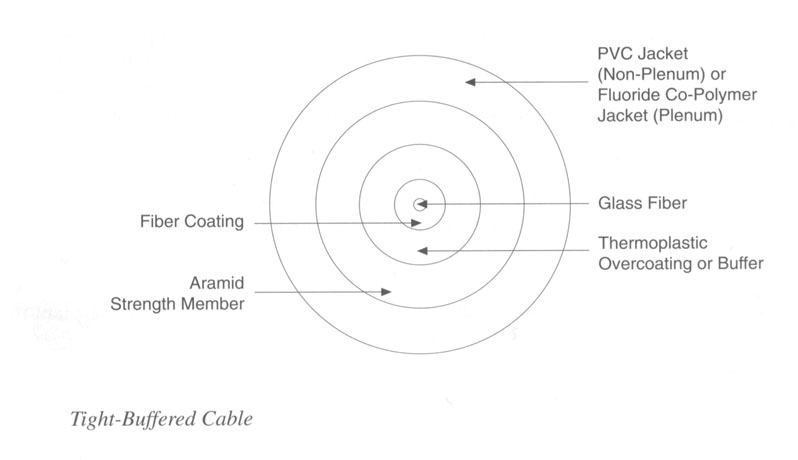
The use of fiber-optics was generally not
available until 1970 when Corning Glass Works
was able to produce a fiber with a loss of 20
dB/km. It was recognized that optical fiber
would be feasible for telecommunication
transmission only if glass could be developed
so pure that attenuation would be 20dB/km or
less. That is, 1% of the light would remain after
traveling 1 km. Today's optical fiber attenuation
ranges from 0.5dB/km to 1000dB/km
depending on the optical fiber used.
Attenuation limits are based on intended
application.
The applications of optical fiber
communications have increased at a rapid
rate, since the first commercial installation of a
fiber-optic system in 1977.
Telephone companies began early on,
replacing their old copper wire systems with
optical fiber lines. Today's telephone
companies use optical fiber throughout their
system as the backbone architecture and as
the long-distance connection between city
phone systems.
Cable television companies have also began
integrating fiber-optics into their cable systems.
The trunk lines that connect central offices have
generally been replaced with optical fiber.
Some providers have begun experimenting
with fiber to the curb using a fiber/coaxial
hybrid. Such a hybrid allows for the integration
of fiber and coaxial at a neighborhood location.
This location, called a node, would provide the
optical receiver that converts the light impulses
back to electronic signals. The signals could
then be fed to individual homes via coaxial
cable.
Local Area Networks (LAN) is a collective
group of computers, or computer systems,
connected to each other allowing for shared
program software or data bases. Colleges,
universities, office buildings, and industrial
plants, just to name a few, all make use of
optical fiber within their LAN systems.
Power companies are an emerging group that
have begun to utilize fiber-optics in their
communication systems. Most power utilities
already have fiber-optic communication
systems in use for monitoring their power grid
systems.
Fiber
by John MacChesney - Fellow at Bell
Laboratories, Lucent Technologies
Some 10 billion digital bits can be transmitted
per second along an optical fiber link in
a commercial network, enough to carry tens of
thousands of telephone calls. Hair-thin fibers
consist of two concentric layers of high-purity
silica glass the core and the cladding, which
are enclosed by a protective sheath. Light rays
modulated into digital pulses with a laser or
a light-emitting diode move along the core
without penetrating the cladding.
The light stays confined to the core because the
cladding has a lower refractive index—
a measure of its ability to bend light.
Refinements in optical fibers, along with the
development of new lasers and diodes, may
one day allow commercial fiber-optic networks
to carry trillions of bits of data per second.
Total internal refection confines light within
optical fibers (similar to looking down a mirror
made in the shape of a long paper towel tube).
Because the cladding has a lower refractive
index, light rays reflect back into the core if they
encounter the cladding at a shallow angle (red
lines). A ray that exceeds a certain "critical"
angle escapes from the fiber (yellow line).

STEP-INDEX MULTIMODE FIBER
has a large core, up to 100 microns in
diameter. as a result, some of the light rays that
make up the digital pulse may travel a direct
route, whereas others zigzag as they bounce off
the cladding. These alternative pathways cause
the different groupings of light rays, referred to
as modes, to arrive separately at a receiving
point. The pulse, an aggregate of different
modes, begins to spread out, losing its
well-defined shape. The need to leave spacing
between pulses to prevent overlapping limits
bandwidth that is, the amount of information that
can be sent. Consequently, this type of fiber is
best suited for transmission over short
distances, in an endoscope, for instance.
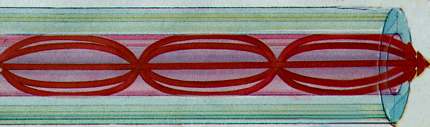
GRADED-INDEX MULTIMODE FIBER
contains a core in which the refractive index
diminishes gradually from the center axis out
toward the cladding. The higher refractive index
at the center makes the light rays moving down
the axis advance more slowly than those near
the cladding. Also, rather than zigzagging off
the cladding, light in the core curves helically
because of the graded index, reducing its travel
distance. The shortened path and the higher
speed allow light at the periphery to arrive at a
receiver at about the same time as the slow but
straight rays in the core axis. The result: a
digital pulse suffers less dispersion.
SINGLE-MODE FIBER
has a narrow core (eight microns or less), and
the index of refraction between the core and the
cladding changes less than it does for
multimode fibers. Light thus travels parallel to
the axis, creating little pulse dispersion.
Telephone and cable television networks install
millions of kilometers of this fiber every year.
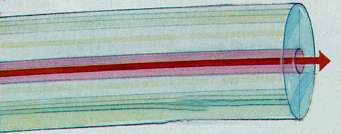
BASIC CABLE DESIGN
1 - Two basic cable designs are:
Loose-tube cable, used in the majority of outside-plant installations in North America, and tight-buffered cable, primarily used inside buildings.
The modular design of loose-tube cables typically holds up to 12 fibers per buffer tube with a maximum per cable fiber count of more than 200 fibers. Loose-tube cables can be all-dielectric or optionally armored. The modular buffer-tube design permits easy drop-off of groups of fibers at intermediate points, without interfering with other protected buffer tubes being routed to other locations. The loose-tube design also helps in the identification and administration of fibers in the system.
Single-fiber tight-buffered cables are used as pigtails, patch cords and jumpers to terminate loose-tube cables directly into opto-electronic transmitters, receivers and other active and passive components.
Multi-fiber tight-buffered cables also are available and are used primarily for alternative routing and handling flexibility and ease within buildings.
In a loose-tube cable design, color-coded plastic buffer tubes house and protect optical fibers. A gel filling compound impedes water penetration. Excess fiber length (relative to buffer tube length) insulates fibers from stresses of installation and environmental loading. Buffer tubes are stranded around a dielectric or steel central member, which serves as an anti-buckling element.
The cable core, typically uses aramid yarn, as the primary tensile strength member. The outer polyethylene jacket is extruded over the core. If armoring is required, a corrugated steel tape is formed around a single jacketed cable with an additional jacket extruded over the armor.
Loose-tube cables typically are used for outside-plant installation in aerial, duct and direct-buried applications.
With tight-buffered cable designs, the buffering material is in direct contact with the fiber. This design is suited for "jumper cables" which connect outside plant cables to terminal equipment, and also for linking various devices in a premises network.
Multi-fiber, tight-buffered cables often are used for intra-building, risers, general building and plenum applications.
The tight-buffered design provides a rugged cable structure to protect individual fibers during handling, routing and connectorization. Yarn strength members keep the tensile load away from the fiber.
As with loose-tube cables, optical specifications for tight-buffered cables also should include the maximum performance of all fibers over the operating temperature range and life of the cable. Averages should not be acceptable.
Connector Types
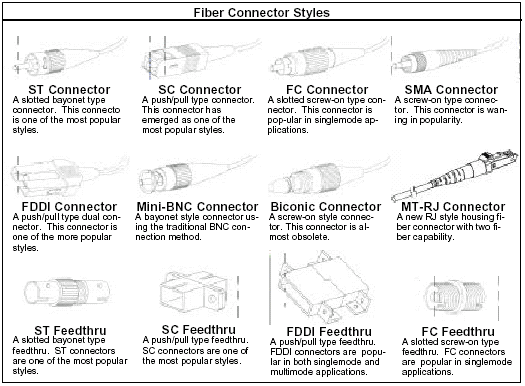
Gruber Industries
cable connectors
here are some common fiber cable types